1. What is an Earthquake?
Earthquakes occur when rock ruptures (breaks) along a fault. A fault is a large planar crack through a body of rock along which there has been movement or shifting. Earthquakes cause rocks on one side of a fault to move relative to the rocks on the other side. The violent shaking felt during an earthquake is not just the rocks slipping past each other but is the rocks vibrating as they release stored up energy. This is like plucking a guitar string; it does not just do one jolt and stop, but instead vibrates sending out sound waves.
1.1 Elastic Rebound Theory
The Elastic Rebound Theory explains where the energy released during an earthquake and the associated shaking come from.
Rocks might seem rigid, but when stress is applied, they may stretch or squish. If there hasn’t been too much stretching, a rock will snap back to its original shape once the stress is removed. Deformation that is reversible like this is called elastic deformation. This is the same principle as a hair elastic – you can stretch it to fit around a ponytail, but once you remove it again, it snaps back to its original, un-stretched shape. The deformed/stretched rock stores a lot of energy, just like a stretched elastic stores a lot of energy – and the more energy it is storing, or the further you have stretched the elastic, the more energy will be released when it snaps back to its original shape. This snapping back is called elastic rebound.
Rocks that are stressed beyond their ability to stretch can rupture – just like if you pull a hair elastic too far, it will break. When this happens, the part of the rock that was stretched too far breaks, but the rest of the rock snaps back to its original shape (just like the rest of the hair elastic will still be a nice stretchy elastic like it always was, just now with a broken part). All the energy that was stored up in the rocks as they were being deformed and stretched gets released and spreads out causing the rock to vibrate, and this is what causes the shaking during an earthquake.
Figure 1B.1.1 (top) shows this sequence of events. Stress is applied to a rock and deforms it. The deformed rock ruptures, forming a fault. After rupturing, the rock above and below the fault snaps back to the shape it had before deformation.
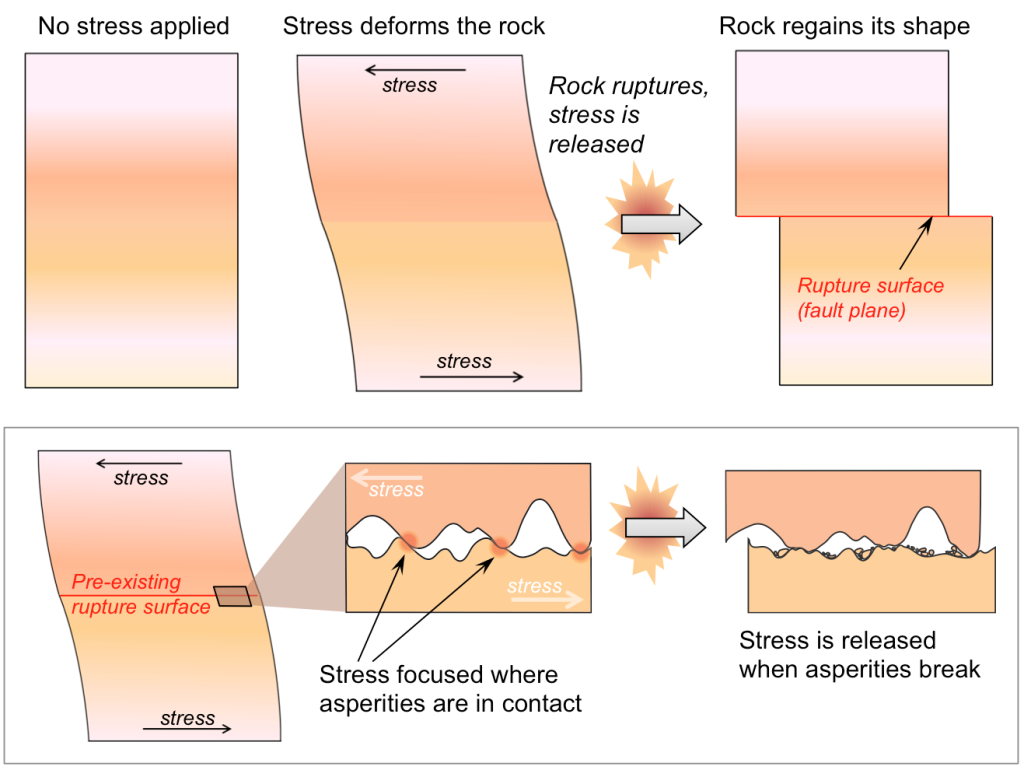
Ruptures can also occur along pre-existing faults (Figure 1B.1.1 bottom). The rocks on either side of the fault are locked together because bumps along the fault, technically called asperities, prevent the rocks from moving relative to each other. When the stress is great enough to break the asperities, the rocks on either side of the fault can slide again. While the rocks are locked together, stress can cause elastic deformation. When asperities break and release the stress, the rocks undergo elastic rebound and return to their original shape.
Here is an animation showing elastic rebound that may be useful for visualizing the process (Source: USGS. Public Domain. Found here.):
1.2 Parts of an Earthquake
While simplified diagrams are useful for illustrating elastic deformation and rupture, they are not fully accurate. The rupture that happens does not necessarily break the rock all the way through, nor does the displacement caused when the rocks move past each other extend along the entire area of the fault. Rupture and displacement only happen along a subsection of a fault, called the rupture surface, although the rupture surface can extend 10s to 100s of kilometers. In Figure 1B.1.2, the rupture surface is the dark pink patch. It takes up only a part of the fault plane (lighter pink). The fault plane is the surface where the fault exists, and where ruptures have happened in the past. Although the fault plane is drawn as being flat, faults are not actually perfectly flat.
The exact location on the fault plane where the rupture happens (i.e., where all that stored energy gets released from initially) is called the hypocenter or focus of the earthquake (Figure 1B.1.2, right). The location on Earth’s surface directly above the hypocenter is the epicenter of the earthquake, i.e., the map location we give to an earthquake.
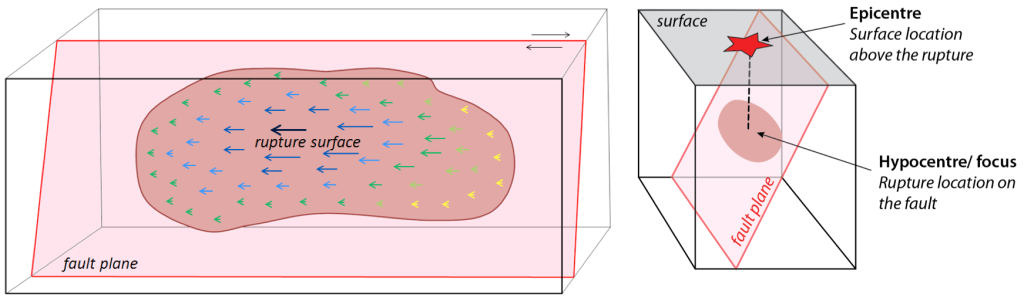
Within the rupture surface, the amount of displacement varies. In Figure 1B.1.2, the larger arrows indicate where there has been more displacement, and the smaller arrows where there has been less. Beyond the edge of the rupture surface there is no displacement at all. Notice that this particular rupture surface does not extend to the land surface of the diagram.
The size of a rupture surface and the amount of displacement along it will depend on several factors, including the type and strength of the rock, and the degree to which the rock was stressed beforehand. The magnitude of an earthquake will depend on the size of the rupture surface and the amount of displacement.
A rupture does not occur all at once along a rupture surface. It starts at a single point and spreads rapidly from there. Figure 1B.1.2 illustrates a case where rupturing starts at the heavy blue arrow in the middle (this would be the hypocenter/focus, or point of rupture initiation), then continues through the lighter blue arrows. The rupture spreads to the left side (green arrows), then the right (yellow arrows).
Depending on the extent of the rupture surface, the propagation of failures (incremental ruptures contributing to making the final rupture surface) from the hypocenter outward is typically completed within seconds to several tens of seconds. The hypocenter is not necessarily in the center of the rupture surface; it may be close to one end, near the top, or near the bottom.
Check your understanding: Basics of earthquakes
Earthquakes do not usually occur in isolation. There is often a sequence of smaller earthquakes before a larger one, and then progressively smaller earthquakes after. The largest earthquake in the series is the mainshock. The smaller ones that come before are foreshocks, and the smaller ones that come after are aftershocks. These descriptions are relative: for example, the strongest earthquake in a series is classified as the mainshock, but if another even bigger one were to occur after it, the bigger one would be called the mainshock, and the earlier one would be reclassified as a foreshock.
Aftershocks and foreshocks are the result of stresses shifting across the area as rocks adjust and move during earthquakes. This adjusting can take pressure off some areas but increase pressure in other areas along the fault causing subsequent earthquakes.