4. The Carbon Cycle
Long term climate on the planet is intimately linked with greenhouse gas levels in the atmosphere, and of these gases carbon dioxide and methane, the two carbon-based gases, have been determined to be important. How and why these gases build up in the atmosphere is a function of the carbon cycle.
The carbon cycle is the natural cycling or movement of carbon on the planet between living organisms, soils, rocks, water, and the atmosphere. It involves interactions between all of Earth’s spheres. Much like the hydrologic cycle, the total amount of carbon in the system is constant, but where in the carbon cycle any particular carbon atom is located shifts continuously. As it cycles, there are places where the carbon “hangs out”, these are termed reservoirs, and processes that move carbon from one reservoir to the next, these are called fluxes.
While the details of the carbon cycle are quite complex, it can be simplified by examining just the major reservoirs and fluxes. Rocks and sediments are the largest reservoir, followed by the oceans, fossil fuels, soils, terrestrial vegetation, and the atmosphere. Figure 3A.4.1 illustrates the relative sizes of each of these to visually represent just how much larger the rock and sediment reservoir is compared to all other reservoirs. Note that these are all to scale with the exception of rocks and sediments; this reservoir would need to be 100 times larger to be to scale.
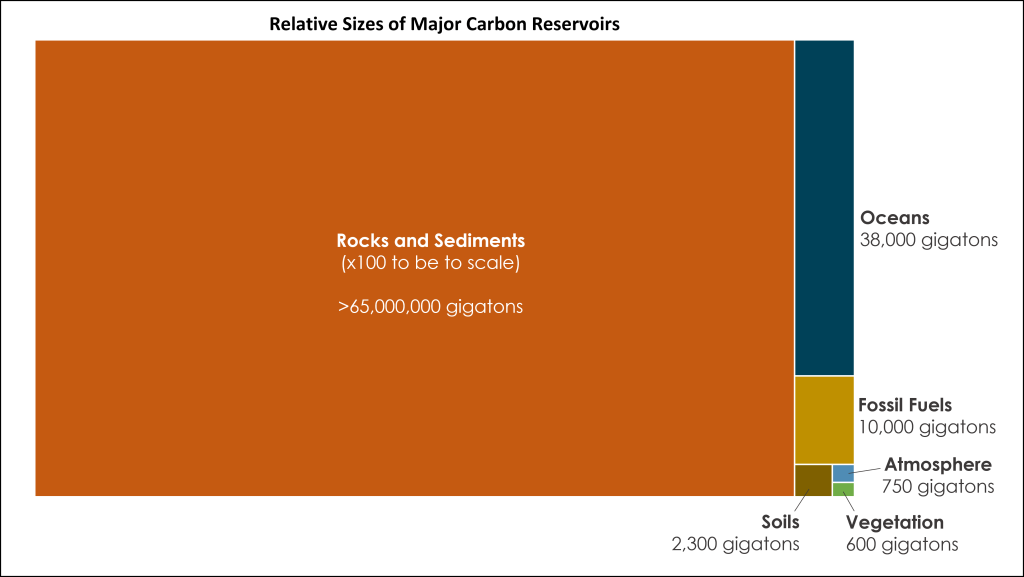
Most of these reservoirs could be subdivided and discussed in more detail. For example, “rocks and sediments” could be subdivided into ocean sediments, terrestrial sediments, lithospheric rocks, and mantle rocks, as there are fluxes that transfer carbon between all of these various parts of the larger reservoir. But, for this discussion of the carbon cycle these sub-reservoirs will be lumped together into the main reservoirs as given in Figure 3A.4.1.
The atmospheric reservoir is so small compared to most of the others. Why do we focus on it for climate change?
While the atmosphere is among the smallest of the major reservoirs, it is important because of the dominant role of carbon dioxide and methane in the greenhouse effect. This means the carbon contained within it is especially important to climate despite its relatively small size. However, while carbon in the atmosphere gets discussed specifically, it is part of a cycle and how that carbon moves into and out of the atmosphere (i.e., the carbon cycle) is actually the larger context for the discussion on climate change. This means all reservoirs and fluxes are important!
When fluxes move carbon out of one reservoir, they transfer it into another reservoir. The carbon cycle can be split into two sub-cycles that move carbon through the system at different rates, the slow carbon cycle and the fast carbon cycle.
4.1 The Slow Carbon Cycle
Slow carbon cycling involves the flux of carbon into and out of rocks and sediments through chemical reactions and plate tectonic activity. The slow carbon cycle moves carbon out of the atmosphere and keeps it out for thousands to many millions of years by cycling it into rocks and sediments, locking it away in fossil fuels, or carrying it deep into the ocean.
In the atmosphere, carbon combines with water to form carbonic acid, creating weakly acidic rainwater that chemically weathers rocks, dissolving them and releasing ions such as calcium, magnesium, and sodium, along with dissolved carbon, which are carried by rivers to the oceans. In the oceans, calcium ions combine with carbonate ions in the water (calcium and oxygen ion, CO32-) to form calcium carbonate, which is the main shell building material for organisms such as shellfish (mussels, clams, etc.), corals, and plankton. When these organisms die, their hard shells sink and become sediment on the ocean floor. These sediments can then be solidified into rocks and eventually subducted into the mantle to add to the reservoir of carbon in the mantle. From there, the carbon can be incorporated in carbon dioxide that is released back to the atmosphere through a volcanic eruption. This is just one example of the pathway a carbon atom could take through the slow carbon cycle. This pathway could keep carbon from cycling back into the atmosphere for several hundred million years.
An alternative pathway in the slow carbon cycle is plants removing CO2 from the atmosphere through photosynthesis and incorporating this carbon as plant material, then dying and accumulating in an environment that turns them into coal over the course of millions of years before being burned and releasing that CO2 back to the atmosphere.
Yet another pathway would be CO2 dissolved from the atmosphere into the surface ocean and then being transferred into deep ocean waters where it could flow with deep thermohaline circulation currents before being brought back to the surface and outgassed from the surface ocean back to the atmosphere. This would be a faster pathway than the previous two examples, but a full trip through the ocean conveyor belt still requires about 1,000 years.
4.2 The Fast Carbon Cycle
The fast carbon cycle transfers carbon in and out of the atmosphere over much shorter time frames, measurable over a human lifetime. The fluxes involved in fast carbon cycling revolve around biological and chemical processes that transfer carbon between the atmosphere, vegetation, soils, and the oceans.
Carbon dioxide is removed from the atmosphere by plants during photosynthesis and used for energy and growth. Plants also respire, or breathe, and release CO2 into the atmosphere. Carbon that is turned into plant material can be eaten by other animals who also breathe and release CO2 to the atmosphere. Carbon dioxide is also released from living organisms once they die and decay. CO2 moving through the food chain and living organisms in these ways are travelling fast carbon cycle pathways.
Another example of a fast carbon cycle pathway begins similarly to the first slow carbon cycle example where carbon combined with rainwater to create carbon acid that falls on the ground. This dissolved carbon can be transported by rivers to the oceans where instead of being taken up as shell material it outgasses from the surface oceans back to the atmosphere.
Figure 3A.4.2 below shows a simplified diagram of the carbon cycle with some of the major fluxes labeled.
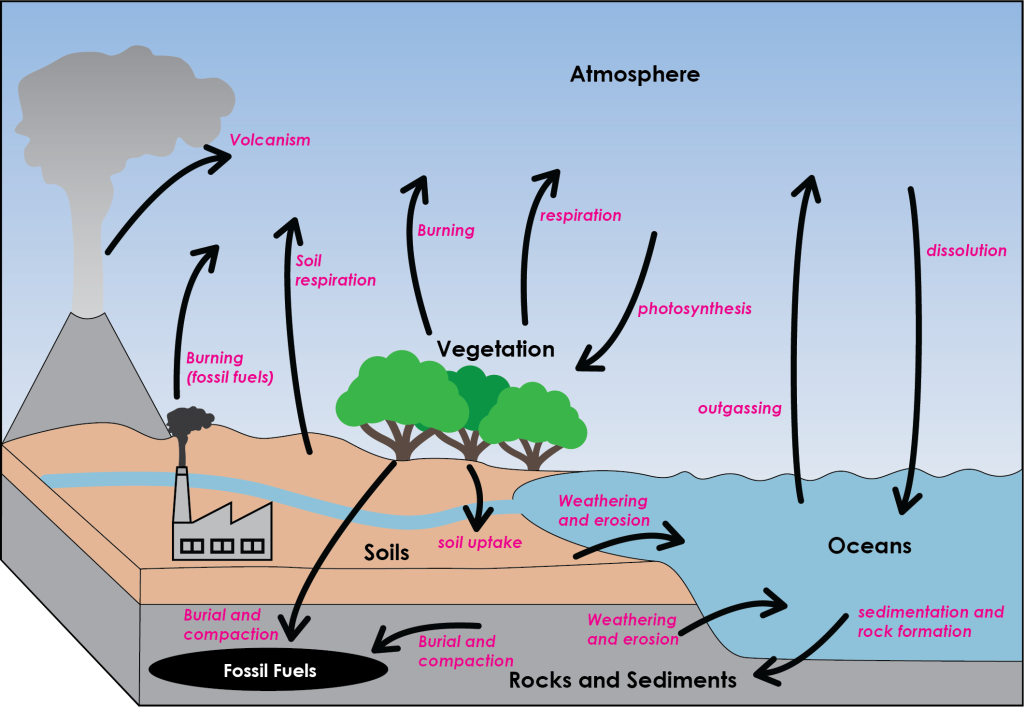
4.3 Balancing the Carbon Cycle
Over the long term, hundreds of thousands of years, movement of carbon between reservoirs is in equilibrium such that the reservoir sizes stay about constant. However, on shorter time scales, hundreds to thousands of years, carbon can become “overloaded” in certain reservoirs leading to temperature fluctuations that can influence glacial and interglacial climates on the planet. This overloading occurs when something causes a flux to change. For example, if a flux increases and starts moving carbon into a reservoir faster than fluxes are removing carbon from that reservoir, the reservoir will grow while another shrinks (Figure 3A.4.3). This happens when the cycle is not in balance.
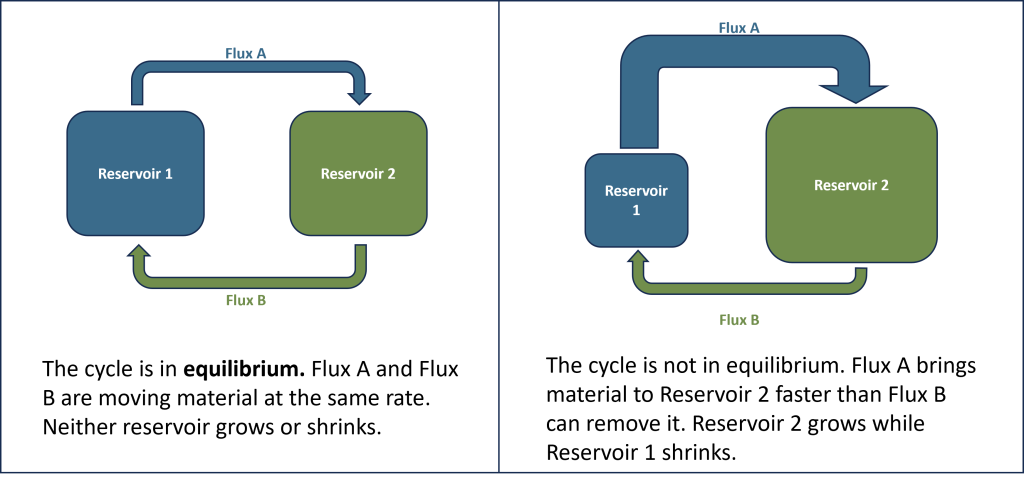
If the carbon cycle is not in balance, it can influence climate by amplifying the effects of other climate forcing mechanisms. For example, increased episodes of volcanic activity on the planet, such as the creation of large igneous provinces (continental flood basalts) could increase the flux of carbon contained within the mantle (rocks and sediments reservoir) into the atmosphere, thereby increasing the greenhouse effect. Tectonic activity that generates mountain building events, such as the formation of the Himalayas, or the creation of supercontinents in Earth’s past, leads to increased erosion of rocks and pulls more carbon out of the atmosphere (this is called carbon drawdown) creating cooling. Over time, these perturbations balance again as the volcanic activity stops or erosion slows down and the rest of the system can catch up and bring everything back into equilibrium.
A change we are currently experiencing on the planet is a change in the amount of carbon being stored in the oceans. As increased carbon emissions have put more carbon into the atmosphere, the oceans have responded by increasing their absorption to keep the system in equilibrium. However, warmer water is not capable of holding as much carbon dioxide as colder water, so while the absorption of carbon into the oceans initially increased to try to equilibrate with rising atmospheric concentrations, that absorption rate is now slowing down as water temperatures have risen.
Current changes to the carbon cycle are a result of human activities like fossil fuel burning and land use changes. The increased emissions of greenhouse gases from these human activities are not balanced by the natural fluxes removing carbon from the atmosphere. In Figure 3A.4.4, natural fluxes of carbon into and out of the atmosphere are labeled in dark blue with values showing the amount of carbon moved by that flux every year in gigatons (Gt/y). Values in red are the flux amounts due to humans, also in Gt/y. The natural fluxes in and out of the atmosphere are balanced leading to no net change in the atmospheric reservoir. This represents the state of the carbon cycle pre-industrialization. The changes to fluxes due to human activity are not balanced and lead to an overall increase in atmospheric carbon of 4 Gt/y.
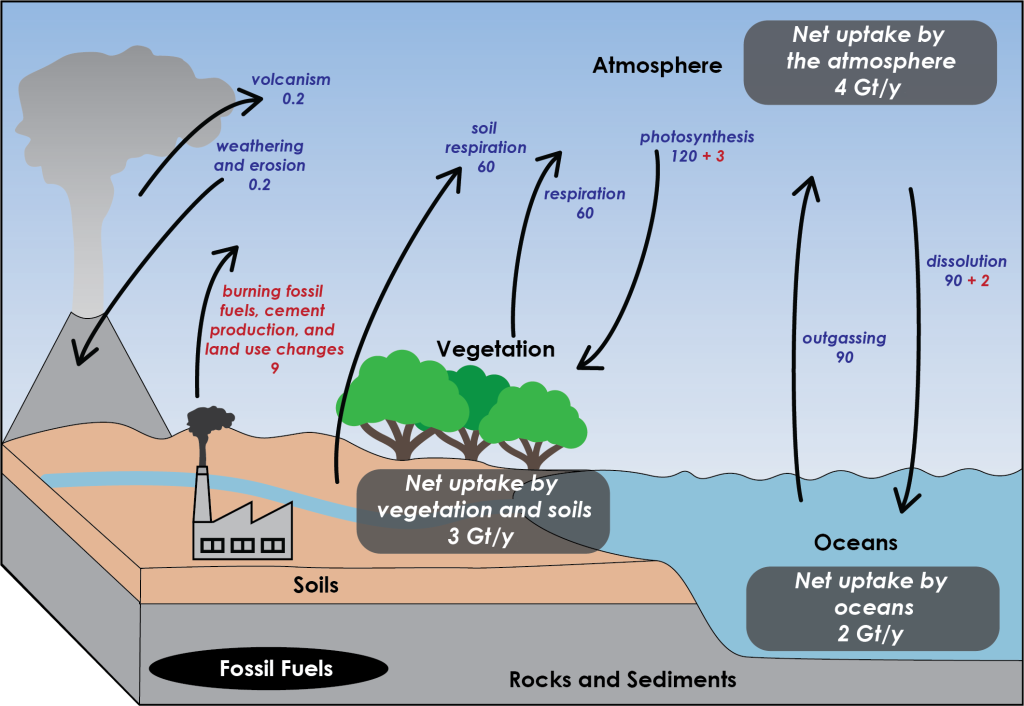
The alterations to the carbon cycle from human activity has resulted in an accelerating increase of carbon within the atmospheric reservoir. Unless the increased flux of carbon to the atmosphere either slows down and stops, or the fluxes removing carbon from the atmosphere speed up, the carbon cycle will remain out of balance.
Check your understanding: The Carbon Cycle
References
NASA Earth Observatory. (n.d.). The carbon cycle. https://earthobservatory.nasa.gov/features/CarbonCycle