2. Earth’s Energy Budget
The flows of energy into and out of Earth’s system is called Earth’s energy budget. It is the balance between energy entering Earth’s system and energy flowing back out to space from Earth. The sun is the source of incoming energy for Earth’s climate; it warms the Earth, powers photosynthesis, drives evaporation and melting. However, as the Earth is a sphere, the sun does not heat the Earth evenly and other processes are required to transfer heat from warmer to colder regions.
The sun is incredibly hot, roughly 5,500 degrees Celsius or 10,000 degrees Fahrenheit, and it transmits this energy (solar radiation) to Earth. At these temperatures, most of this energy is in the visible light and near infrared light wavelengths. The rate at which this energy is arriving to Earth’s surface per unit area is called solar irradiance. The solar irradiance varies across the Earth both throughout the day and depending on distance from the equator. When an area on the planet is facing away from the sun, i.e., nighttime, it is not receiving solar energy. Equatorial regions have a higher solar irradiance than polar regions because sunlight is more direct at the equator, therefore the energy is greater per unit of area at the equator than at the poles (Figure 3A.2.1). The maximum solar irradiance on the planet is at a location near the equator at midday when the sun is at its highest in the sky and therefore sunlight is hitting the Earth most directly.
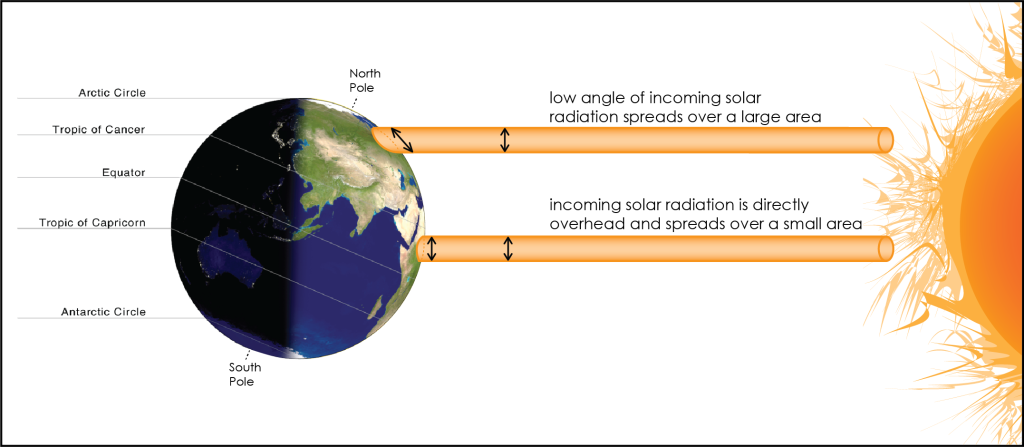
Because the Earth’s rotational axis is tilted relative to its orbit around the sun (this is why there are seasons), the amount of solar irradiance received also varies depending on the time of year. At points in the mid latitudes, let’s say Minnesota where 45°N runs right through Minneapolis, the solar energy received is far greater in the summertime than in the wintertime. This is felt by warmer temperatures (much warmer temperatures!) and is seen with many more hours of daylight in the summer than in the winter. This difference is even more extreme at polar locations where in the wintertime the sun is not visible at all; there is no sunrise or sunset for 11 weeks at the north and south poles during their winters.
In addition to different areas receiving different amounts of solar energy, the different surface materials in these areas alter how much of that solar energy is absorbed or reflected. The reflectivity of a surface is called its albedo and will be discussed more in the next section. Generally, materials that are light in color, such as ice and snow found at the poles, reflect more energy while materials that are dark in color, like a forest or open ocean, absorb more energy.
The combined effects of solar irradiance and albedo differences mean equatorial regions receive and absorb more solar energy than polar regions. However, because of the high reflectivity of ice and snow at the poles, polar regions actually reflect and emit more energy than they receive, leading to an overall surplus of heat energy near the equator and a deficit at the poles. Despite this, the equator does not keep heating up and the poles do not keep getting colder, instead a transfer of heat balances the energy difference away from the equator toward the poles and from Earth’s surface and lower atmosphere back into space (Figure 3A.2.2).
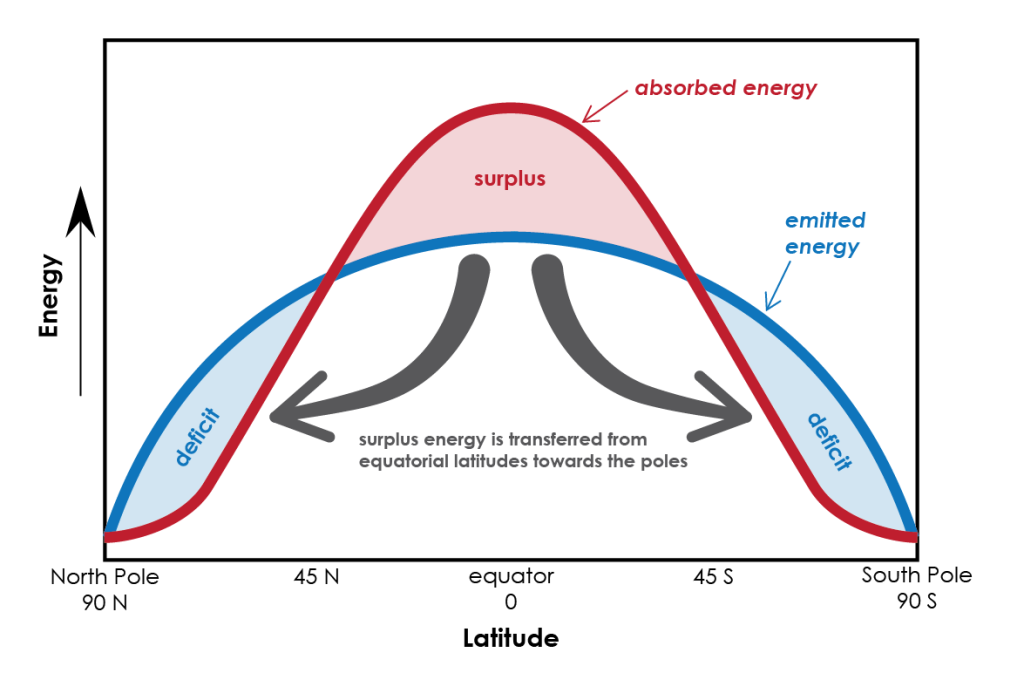
The reason Earth’s temperature remains relatively stable over long periods of time is because Earth’s energy budget is in equilibrium. The amount of incoming energy is equal to the amount of outgoing energy in the system and this energy moves between three different locations: energy outside the atmosphere, energy within the atmosphere, and energy at the Earth’s surface (Figure 3A.2.3).
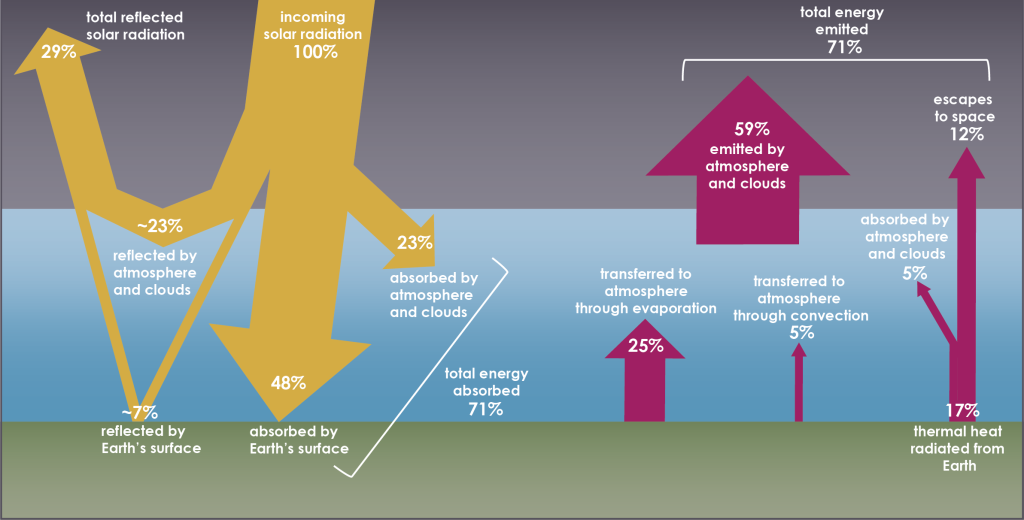
As solar radiation arrives from outside the atmosphere, it interacts with the atmosphere where about 29% is reflected (~23% is reflected directly by the atmosphere, and ~7% is reflected from Earth’s surface). About 23% of the incoming solar radiation is absorbed by the atmosphere and the remaining 48% makes it through the atmosphere to be absorbed by Earth’s surface. In all, a total of 71% of solar energy enters the Earth system (atmosphere and surface). As the surface, not the atmosphere, receives the largest percentage of incoming solar energy, this is where the most heating occurs.
However, most of the outflow of energy back into space comes from the atmosphere (59% of total initial solar energy), which means there has to be a way to transfer heat from the surface to the atmosphere to be released. The transfer of the 48% of heat from the surface to atmosphere occurs in three ways. Evaporation moves 25% of energy to the atmosphere and 5% is moved through convection. The remaining 17% is thermal (heat) energy that is radiated back out from the surface where 5% is absorbed by the atmosphere and 12% makes it through the atmosphere to escape into space. The atmosphere and clouds release another 59% of energy, which added to the 12% that escaped from Earth’s surface makes a total of 71% of energy released to space; the same amount that entered at the start. In this way, Earth’s energy budget is in equilibrium.
Check your understanding: Earth’s energy budget
References
NASA Earth Observatory. (n.d.). Climate and Earth’s energy budget. https://earthobservatory.nasa.gov/features/EnergyBalance