2. Pre-Cenozoic Ice Ages
2.1 Huronian Ice Age
The Huronian Ice Age was between 2.4 and 2.1 billion years ago. Rocks of this age are rare so it is difficult to determine the full intensity and extent of this ice age, but some researchers suggest ice coverage may have extended into the low latitudes making this a Snowball Earth event like the later Cryogenian Ice Age.
Regardless of the extent of ice coverage, major changes on the planet leading up to this time triggered the onset of glaciation. Our knowledge of early life on Earth is rapidly changing, but by at least 3.7 billion years ago there was life in the form of microscopic organisms. The atmosphere at this time had much more methane and CO2 than today and these organisms relied on chemosynthesis or other biologic energy pathways to survive but did not produce oxygen through photosynthesis. The atmosphere and oceans at this time were largely anoxic (without oxygen). Photosynthetic cyanobacteria had evolved by 2.7 billion years ago and with their presence atmospheric and ocean chemistry began to change. They are responsible for what is termed the Great Oxidation Event (GOE), which occurred between 2.3 to 2.5 billion years ago (Figure 3B.2.1). Photosynthesis added oxygen to both the atmosphere and the oceans, reducing the concentration of carbon dioxide and even more importantly, greatly reducing the methane concentration in the atmosphere. This weakened the strong greenhouse conditions that had existed on the planet, lowering global temperatures, and sending the planet into the Huronian Ice Age.
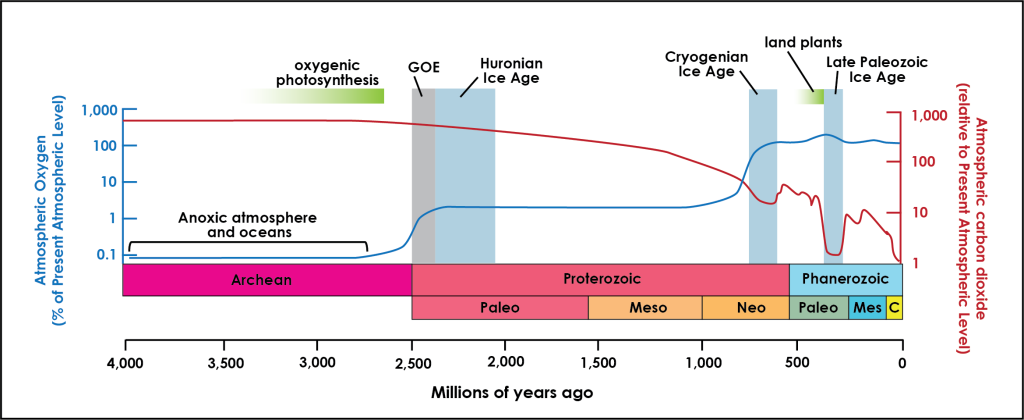
Leading up to the GOE, the anoxic environment of the oceans allowed iron to be present as a dissolved ion in ocean water. As photosynthesis changed ocean chemistry and oxygenated the water the dissolved iron bonded with oxygen to create iron oxides. This is the same process that occurs when you leave iron outside to rust; oxygenating the oceans effectively created rust! Iron oxide is not soluble in water, so it precipitated (formed a solid) that settled on the sea floor as a layer of iron rich sediment.
Oxygenating the oceans was not completed in one attempt. It took time to build oxygen levels to the persistent levels of today, and throughout this time oxygen levels would rise and fall again. When oxygen levels were high, dark colored iron-rich sediments would accumulate and when oxygen levels were low, iron-poor sediments that were dominantly red colored chert, a microcrystalline form of quartz, would accumulate. In this way layers of dark iron-rich and red iron-poor sediments built up over time on the ocean floor. These are known as banded iron formations, or BIFs (Figure 3B.2.2, left). Once the dissolved iron in the oceans had all been oxidized and the oceans had become fully oxygenated, there was no longer a mechanism for creating BIFs. As a result, all BIFs on the planet are old, with the vast majority of them forming between 2.8 and 1.8 Ga, associated with the GOE. Another pulse of oxygenation later in the Neoproterozoic, possibly associated with the Cryogenian Ice Age, yielded minor amounts of BIF that are about 750 Ma. The youngest BIFs found on the planet are about 520 Ma, but are very rare and highly localized, suggesting the conditions for creating BIFs remained in only small pockets of ocean by this time. No banded iron has formed since this time.
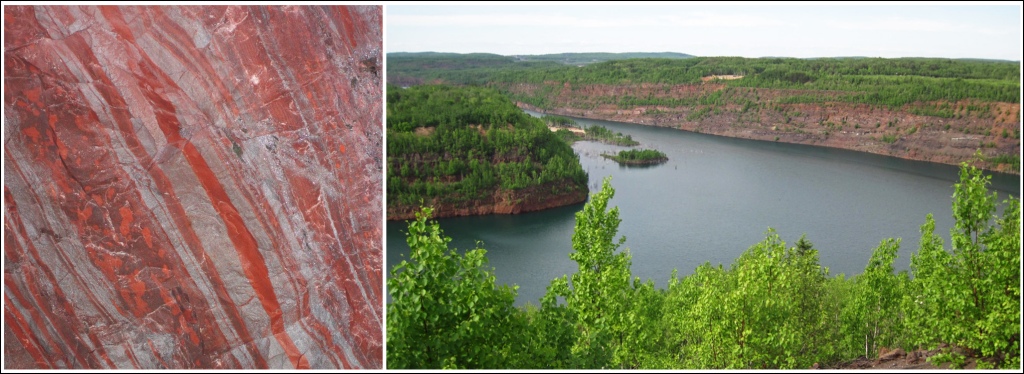
These are the rocks found in the Iron Range of Northern Minnesota (and Michigan) (Figure 3B.2.2, right). Traditionally iron mining was the main economic driver through this area of Minnesota but has gone through boom-and-bust cycles. Bob Dylan, who grew up on the Iron Range, wrote about the dying economy and way of life in the area as a result of mine closures in his 1963 song “North Country Blues”. More recently outdoor recreational tourism has increased at the same time iron mining has waned. The area is a wealth of iron and other metal resources that are widely needed and used but also home to vast natural areas like the Boundary Waters Canoe Area that are susceptible to environmental degradation brought about by mining activities. As a result, mining in this area has become a source of tension between mining supporters and mining detractors including many environmental groups.
2.2 Cryogenian Ice Age
The Cryogenian Ice Age from about 720 to 630 Ma is commonly referred to as the “Snowball Earth”. Continental landmasses were joined into the supercontinent Rodinia during this time, with much of the landmass distributed from mid to low latitudes crossing the equator. There is evidence of ice sheets across Rodinia, even at equatorial latitudes, during the Cryogenian Ice Age suggesting the entire planet was covered in ice, hence the name “Snowball Earth”. There is debate over just how frozen the planet was and whether it was truly a “snowball” or more of a “slushball” where low latitude ice was thin and slushy with areas of open ocean water. Regardless, the Cryogenian Ice Age was the most extensive glaciation the Earth has experienced.
As Rodinia began rifting apart, large expanses of continental flood basalts were emplaced between 850 and 720 Ma. This started the cooling process by releasing volcanic sulfur aerosols and increasing Earth’s albedo. Further cooling continued as extensive weathering and erosion of the basalts pulled carbon dioxide out of the atmosphere. Given their mineral composition, the weathering of basalts pulls more carbon dioxide out of the atmosphere than does the weathering of typical andesitic or granitic continental rocks. In addition, the presence of basalt along the equator where increased rainfall leads to increased weathering worked together to pull CO2 out of the atmosphere faster than outgassing from volcanism could replace it. The atmospheric reduction of CO2 at this time can be seen in Figure 3B.2.1. This weakened the greenhouse effect allowing ice to grow starting near the poles where solar irradiance was weakest. As the ice-albedo feedback took over, glaciers extended into equatorial zones turning the planet into a snowball.
The planet did not stay in a perpetual snowball state because volcanism is not controlled by incoming solar energy or albedo feedbacks. Plate tectonics kept grinding away, rifting Rodinia apart with associated volcanism and outgassing of carbon dioxide. With the planet in a snowball state with no exposed rock, the drawdown of CO2 related to weathering would have stopped, allowing the atmosphere to increase in CO2 again, strengthening the greenhouse effect and bringing the planet out of the icehouse conditions.
Check your understanding: Snowball Earth
2.3 Late Paleozoic Ice Age
The third ice age noted on Figure 3B.2.1 is the Late Paleozoic Ice Age from about 360 to 260 Ma and was the longest ice age of the Phanerozoic. The causes of this ice age share commonalities with both Proterozoic ice ages and resulted from a combination of increased photosynthesis and weathering related drawdown of atmospheric CO2.
Land plants first evolved during the Mid-Paleozoic, roughly 500 million years ago, but during the Devonian, the time period leading up to the Late Paleozoic Ice Age, land plants diversified and expanded leading to increased photosynthesis. This is seen as a small “bump” in the oxygen curve on Figure 3B.2.1. Atmospheric oxygen levels reached their highest at 35%. This was a time of giant ferns, extensive forests, and large wetlands (Figure 3B.2.3).

The large forests and extensive plant life increased the carbon reservoir size of vegetation at the expense of atmospheric carbon. This continued into the Carboniferous (the time period following the Devonian), and ultimately the carbon was pulled out of the system long term as the plant life died and was buried in swamps to create the coal we use today.
The rise in plant life and sequestering of carbon in vegetation was accompanied by the formation of the supercontinent Pangea, which grew large mountains and drove atmospheric CO2 drawdown through weathering and erosion. This precipitous drop in atmospheric CO2, easily identifiable in Figure 3B.2.2, greatly weakened the greenhouse effect. The portion of Pangea situated over the southern pole was at an ideal latitude for glaciers to grow in the cooler climate. These glaciers covered large parts of what are now Africa, South America, Australia, and Antarctica. The glacial landforms created during this time, which join up across continents that are now far apart, were an important component of Alfred Wegener’s evidence for continental drift.
References
Berner, R. A. (1999). Atmospheric oxygen over Phanerozoic time. Proceedings of the National Academy of Sciences of the United States of America, 96(20), 10955–10957. https://doi.org/10.1073/pnas.96.20.10955
Condie, K. C. (2022). Earth cycles. In Elsevier eBooks (pp. 197–227). https://doi.org/10.1016/b978-0-12-819914-5.00001-9
Cox, G., Halverson, G. P., Stevenson, R., Vokaty, M., Poirier, A., Kunzmann, M., Li, Z., Denyszyn, S. W., Strauss, J. V., & Macdonald, F. A. (2016). Continental flood basalt weathering as a trigger for Neoproterozoic Snowball Earth. Earth and Planetary Science Letters, 446, 89–99. https://doi.org/10.1016/j.epsl.2016.04.016
Hoffman, P. F., Kaufman, A. J., Halverson, G. P., Schrag, D. P. (1998). A Neoproterozoic Snowball Earth. Science, 281(5381), 1342–1346. https://doi.org/10.1126/science.281.5381.1342
Kopp, R., Kirschvink, J. L., Hilburn, I. A., & Nash, C. Z. (2005). The Paleoproterozoic snowball Earth: A climate disaster triggered by the evolution of oxygenic photosynthesis. Proceedings of the National Academy of Sciences of the United States of America, 102(32), 11131–11136. https://doi.org/10.1073/pnas.0504878102
Kurucz, S., Fralick, P., Homann, M., & Lalonde, S. V. (2021). Earth’s first snowball event: Evidence from the early Paleoproterozoic Huronian Supergroup. Precambrian Research, 365, 106408. https://doi.org/10.1016/j.precamres.2021.106408
Luo, G., Ono, S., Beukes, N. J., Wang, D. T., Xie, S., & Summons, R. E. (2016). Rapid oxygenation of Earth’s atmosphere 2.33 billion years ago. Science Advances, 2(5). https://doi.org/10.1126/sciadv.1600134
Lyons, T. W., Reinhard, C. T., & Planavsky, N. J. (2014). The rise of oxygen in Earth’s early ocean and atmosphere. Nature, 506(7488), 307–315. https://doi.org/10.1038/nature13068
Macdonald, F. A., & Wordsworth, R. (2017). Initiation of Snowball Earth with volcanic sulfur aerosol emissions. Geophysical Research Letters, 44(4), 1938–1946. https://doi.org/10.1002/2016gl072335
Shih, P. M., Occhialini, A., Cameron, J. C., Andralojc, P. J., Parry, M. a. J., & Kerfeld, C. A. (2016). Biochemical characterization of predicted Precambrian RuBisCO. Nature Communications, 7(1). https://doi.org/10.1038/ncomms10382
Warke, M. R., Di Rocco, T., Zerkle, A. L., Lepland, A., Prave, A. R., Martin, A. P., Ueno, Y., Condon, D. J., & Claire, M. (2020). The Great Oxidation Event preceded a Paleoproterozoic “snowball Earth.” Proceedings of the National Academy of Sciences of the United States of America, 117(24), 13314–13320. https://doi.org/10.1073/pnas.2003090117
petm/eocene climate optimum
pleistocene but the start of the Pleistocene ice age or Pleistocene Glaciation, which is commonly just referred to as the “ice age” when people think of ice age is broadly considered about 2.5 million years ago when ice sheet growth began in the northern hemisphere as well.